Medical Applications of Gel
Taking advantage of the similarities between hydrogels and biological tissues, biomedical applications of gels such as next-generation artificial cartilage and regeneration of cartilage tissue in vivo, etc. have been investigated in collaboration with Hokkaido University medical school.
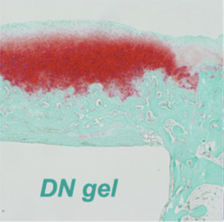
1. Cartilage and Current Joint Replacement
Cartilage tissue can be considered a “natural hydrogel” in which about 5% proteoglycan and 15% type II collagen form a network that retains about 75% water. This high water content enables low friction and high shock absorption in joints. However, since only 1% or so of chondrocytes are present in this tissue, and blood vessels that supply nutrients to the cells hardly pass through, natural healing of cartilage from injury, aging, etc. rarely occurs. Especially in modern society where life expectancy is increasing, almost all elderly people suffer from some degree of arthritis. Currently the most effective treatments involve invasive and complex complete joint replacement surgeries, but there are clinical problems with this approach: for example, the remaining healthy tissue must also be removed, and artificial joints can degrade and require repair after long-term use. Because of these problems, next-generation artificial cartilage that is closer in physical and biological properties to natural tissue are desired for tissue reconstruction. Here, we introduce our research on applying high toughness double network gels (commonly called DN gels), developed in our laboratory, to scaffolds for artificial cartilage and cartilage regeneration. This research was performed in collaboration with Dr. Yasuda of Hokkaido University School of Medicine.
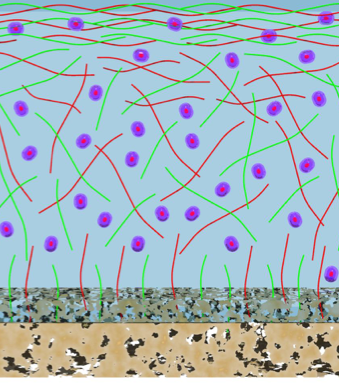
1-1. Potential of Robust Gels for Artificial Cartilage
Soft and wet hydrogels have many similarities with living tissues, but their poor mechanical properties seriously limit their application. Extremely high strength and high toughness hydrogels have been extensively researched by our laboratory and others since the early 2000s, and their mechanical strength has reached a sufficient level for their use as bio-structural materials. Therefore, research on next-generation medical materials have attempted to incorporate these new hydrogels, since they possess properties closer to natural materials, especially compared to traditional hard materials such as metals or ceramics. Clinical application for artificial joints requires gels with high wear resistance and low friction. We made various types of DN gels and performed friction tests by pressing hard alumina spheres into the gel and rubbing repeatedly for over 1,000,000 cycles. The result of these experiments demonstrated that the wear resistance of the prepared DN gels was as good as the high-density polyethylene used in current artificial joints.
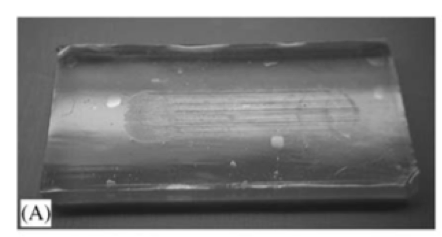
Beyond impressive wear-resistant properties, the calculated coefficient of friction is also comparable to that of polyethylene. These results mean that DN gels have both the high wear resistance and low friction required for current artificial joints. Furthermore, in order to simulate a more comparable testing environment, the same friction tests were performed within a rabbit knee joint, and the results showed that the friction coefficient was even lower when the joint moved. Also, tissue damage on the other joint surface was not observed. Currently, we are promoting joint remedy research with companies and aiming for clinical application, including in peripheral technologies such as establishing a method for bonding gel to bone.
1-2. Regeneration of Cartilage Tissue induced by Robust Gels
Cartilage is a “single-use” tissue, meaning that as it is used, it breaks down, similar to a rubber eraser diminishing with use. Due to this nature, cartilage is known to not be capable of healing independently in the human body. However, during the research aiming to apply DN gels to artificial joints, we unexpectedly discovered that DN gels have properties that stimulate regeneration of cartilage tissue. When a cylindrical DN gel was implanted in a slightly deeper defect created in a rabbit joint, hyaline cartilage tissue begins to form gradually in the gap created by the DN gel from about 2 weeks after surgery, and the defect wass effectively healed in 4 weeks. (corresponding to the red phase in the figure).
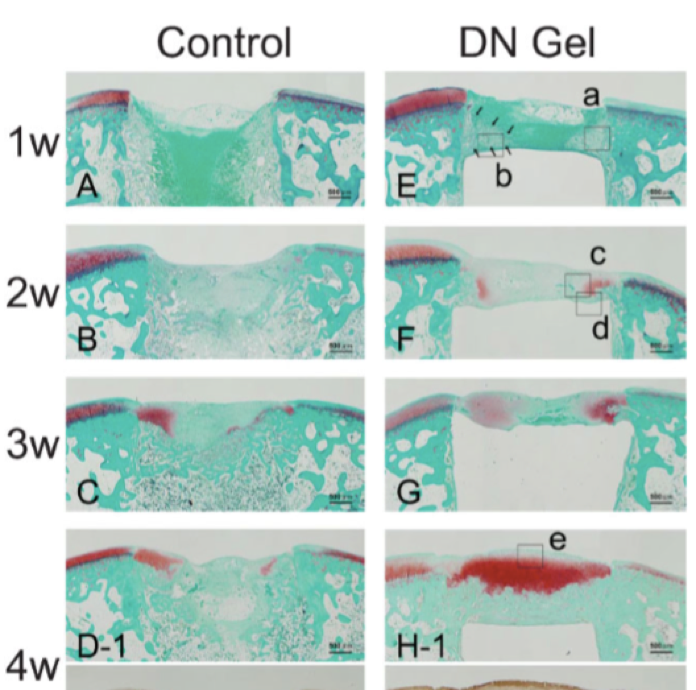
For comparison, when we implanted high density polyethylene in the defect, or if nothing was implanted, no regeneration was observed. The amount of gene expression involved in hyaline cartilage formation and the differentiation to chondrocytes was also extremely high compared to the controls, indicating that DN gels induce cartilage tissue regeneration. This is the first research result in the world demonstrating that cartilage tissue can be regenerated in vivo without cultured cell injections.
2. Biocompatible Gels
It is an important requirement that biomaterials that are implanted in the body must not cause a strong inflammatory response at the implantation site. Inflammation occurs when a type of white blood cell, called a macrophage, digests unwanted or foreign matter in the body. We prepared zwitterionic gels, which contain both positive and negative charges within a single monomer, and evaluated the adhesion of macrophages. The experimental results demonstrate that macrophages do not adhere at all to these types of gels. As a comparison, when we seed macrophages on a polystyrene dish for cell culture, many macrophages adhere.
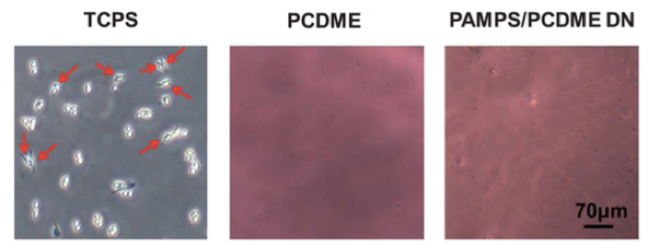
Also, in gels prepared by mixing positively and negatively charged monomers so that the net charge over the whole chain is zero, adhesion of the macrophages was also hardly observed. These results show that it is possible to produce electrically neutral gels that have high antifouling capability and biocompatibility.
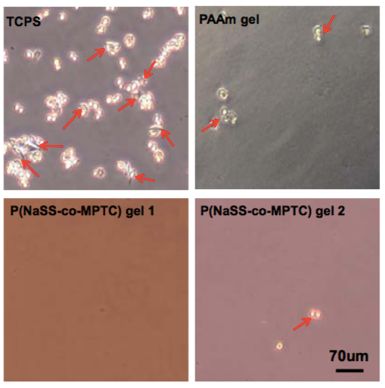
References
- K. Yasuda, J. P. Gong, Y. Katsuyama, A. Nakayama, Y. Tanabe, E. Kondo, M. Ueno, Y. Osada,Biomaterials 2005, 26, 4468-4475.
- K. Arakaki, N. Kitamura, H. Fujiki, T. Kurokawa, M. Iwamoto, M. Ueno, F. Kanaya, Y. Osada, J. P. Gong, K. Yasuda,J Biomed Mater Res A 2010, 93A, 1160-1168.
- K. Yasuda, N. Kitamura, J. P. Gong, K. Arakaki, H. J. Kwon, S. Onodera, Y. M. Chen, T. Kurokawa, F. Kanaya, Y. Ohmiya, Y. Osada,Macromol Biosci 2009, 9, 307-316.
- H. Y. Yin, T. Akasaki, T. L. Sun, T. Nakajima, T. Kurokawa, T. Nonoyama, T. Taira, Y. Saruwatari, J. P. Gong,J. Mater. Chem. B 2013, 1, 3685-3693.
- T. L. Sun, T. Kurokawa, S. Kuroda, A. Bin Ihsan, T. Akasaki, K. Sato, M. A. Haque, T. Nakajima, J. P. Gong,Nat Mater 2013, 12, 932-937.
Related News
-
The Supplementary Cover Art for the manuscript written by Dr. Martin Frauenlob is now published in ACS Macro Letters!
- Awards & Publications
-
[Press Releas] Uprooting cancer: Hydrogel rapidly reverts cancer cells back to cancer stem cells(Hokkaido U Press Release, March 30, 2021)
- Research